CRISPR is a hot topic right now, and rightly so—it is revolutionizing research that relies on editing genes. But what exactly is CRISPR? How does it work? Why is everyone so interested in using it? Today’s blog is a beginner’s guide on how CRISPR works with an overview of some new applications of this technology for those familiar with CRISPR.
Introduction to CRISPR/Cas9
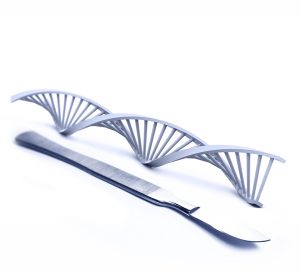
Clustered Regularly Interspaced Short Palindromic Repeats (CRISPR) were discovered in 1987, but it took 30 years before scientists identified their function. CRISPRs are a special kind of repeating DNA sequence that bacteria have as part of their “immune” system against invading nucleic acids from viruses and other bacteria. Over time, the genetic material from these invaders can be incorporated into the bacterial genome as a CRISPR and used to target specific sequences found in foreign genomes.
CRISPRs are part of a system within a bacterium that requires a nuclease (e.g. Cas9), a single guide RNA (sgRNA) and a tracrRNA. The tracrRNA recruits Cas9, while sgRNA binds to Cas9 and guides it to the corresponding DNA sequence of the invading genome. Cas9 then cuts the DNA, creating a double-stranded break that disables its function. Bacteria use a Protospacer Adjacent Motif, or PAM, sequence near the target sequence to distinguish between self and non-self and protect their own DNA.
While this system is an effective method of protection for bacteria, CRISPR/Cas9 has been manipulated in order to perform gene editing in a lab (click here for a video about CRISPR). First, the tracrRNA and sgRNA are combined into a single molecule. Then the sequence of the guide portion of this RNA is changed to match the target sequence. Using this engineered sgRNA along with Cas9 will result in a double-stranded break (DSB) in the target DNA sequence, provided the target sequence is adjacent to a compatible PAM sequence.
Cells have two options for repairing this DSB, and researchers take advantage of both. Non-Homologous End Joining (NHEJ) will simply bring the two ends together. This process is prone to errors that result in a gene knockout. A cell can also use another homologous DNA sequence to serve as a template for repairing the DSB. In order to direct that repair, you can create a DNA molecule with the desired sequence and deliver it to the cell with the other CRISPR/Cas9 components. The cell will then use this sequence as a template and change the sequence of the gene, which could restore a mutated gene to the wild type or create a new mutation.
Pros and Cons of Using CRISPR/Cas9
CRISPR/Cas9 gene editing offers significant advantages over other methods, such as ZNFs and TALENs, which explains its steep rise in popularity. It is relatively quick and easy to engineer the components needed for CRISPR/Cas9 gene editing, so significant training or expertise isn’t necessary. Along with being user-friendly, gene editing via CRISPR/Cas9 is cheaper—ZNFs are about 160x more expensive than using CRISPR/Cas9.
Since the use of CRISPR/Cas9 has experienced such rapid progress, researchers haven’t had time to precisely characterize or optimize its tools. This means that CRISPR/Cas9 can be inefficient and result in off-target mutations. Another concern is that gene edits made with CRISPR/Cas9 cannot be traced as they are with traditional methods, leaving no way of identifying engineered genomes. Furthermore, the accessibility of CRISPR/Cas9 intensifies a host of ethical questions that already exist surrounding genetic modification: Who should be able to use these tools? Where do we draw the line for genetic modification? And, should these tools be used for germ line genomic editing?
Applications of CRISPR/Cas9
Despite these concerns, the drug development community is hopeful that CRISPR/Cas9 can be used as a therapeutic to correct disease-causing mutations and succeed as a tool for gene therapy where previous methods have not. At this point, CRISPR/Cas9 is most effective in rapidly dividing cells and must be applied to cells in vitro. Both circumstances significantly limit the pool of diseases that could potentially benefit from CRISPR/Cas9 in its current state. As a result, many remain skeptical about CRISPR/Cas9’s therapeutic potential.
Although CRISPR/Cas9 is a hot topic in the world of drug development, there are many applications of the technology being used in research. CRISPR/Cas9 is being explored as a new approach to genetically engineering plants and livestock, creating model organisms, and screening for relationships between genes and cell responses. All of these uses rely on the basic CRISPR/Cas9 toolkit mentioned earlier. Some more novel developments of CRISPR/Cas9 involve gene editing at the population level and changing gene expression without permanently altering the DNA sequence.
Gene drives are a way to get an edited gene to spread quickly in a population. In a gene drive, a CRISPR-derived mutation on one chromosome has the ability to copy itself onto its partner chromosome. This copying happens every generation, meaning virtually all offspring will inherit the genetic change. Accelerating the spread of genes in a population could be a very effective method of eliminating disease vectors, invasive plants, or species that are resistant to herbicides or pesticides. For example, a mutation to reduce the number offspring produced could be engineered into a deer tick, causing population collapse of the ticks, as well as parasites they may be carrying—like the bacteria that causes Lyme disease.
Innovations to the CRISPR/Cas9 tools can be used to produce changes in gene expression that are analogous to epigenetic control. Altering Cas9 by disabling its ability to cut can turn off a gene, while adding a protein that modifies gene expression can activate a gene. You can take this a step further and attach a protein to Cas9 that is sensitive to light, producing an “on/off” switch for a gene controlled by light. Manipulating guide RNA to be longer in order to bind to multiple targets along a DNA strand allows the simultaneous activation or inhibition of multiple genes.
While CRISPR/Cas9 is growing rapidly in popularity, caution is necessary to avoid unforeseen, long-term consequences that are irreversible. (See the U.S. National Academies of Sciences, Engineering, and Medicine report recommending against deployment of gene drive modified organisms into the environment.) At the same time, the hope and promise this technology offers as a means to enrich research and deal with previously intractable diseases warrants more exploration and innovation of CRISPR/Cas9 and its potential applications.
Want to see how we have used this technique to build a catalog of available cell lines or get information on how to do it yourself? Learn more
Related Posts
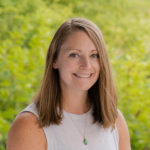
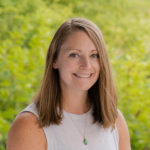
Latest posts by Darcia Schweitzer (see all)
- Cytochrome P450 Inhibition: Old Drug, New Tricks - May 5, 2022
- Firefly Luciferase Sheds Light on Development of New Malaria Treatments - April 5, 2021
- How to Train Your Instrument Service Team in a Pandemic - February 1, 2021
One thoughtful comment