Updated 8/25/2021, 4/29/2024
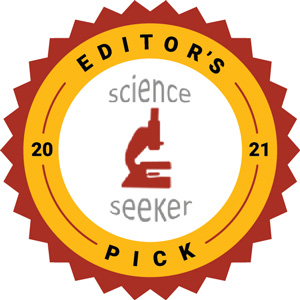
Multiple battles are being fought in the war against the SARS-CoV-2 coronavirus that causes COVID-19. Currently, there are nearly 5,000 clinical trials listed in the World Health Organization (WHO) database, either underway or in the recruiting stage, for vaccines and antiviral drugs. The Moderna mRNA vaccine and Janssen vaccine received emergency use authorization (EUA) from the Food and Drug Administration (FDA) and have since been fully approved; the Pfizer-BioNTech Vaccine (marketed as Comiraty) received FDA approval in August 2021.
Both the Moderna vaccine and Comiraty are mRNA-based, as opposed to most conventional vaccines against established diseases that are protein-based. Typically, the key ingredient in viral vaccines is either part of an inactivated virus, or one or more expressed proteins (antigens) that are a part of the virus. These protein antigens are responsible for eliciting an immune response that will fight future infection by the actual virus. Another approach is to use a replication-deficient viral vector (such as adenovirus) to deliver the gene encoding the antigen into human cells. This method was used for the coronavirus vaccine developed by Oxford University in collaboration with AstraZeneca; phase 3 interim data were announced on the heels of the Pfizer/BioNTech and Moderna announcements. All three vaccines target the SARS-CoV-2 spike protein, because it is the key that unlocks a path of entry into the host cell.
Vaccine Development: Then and Now
Historically, the fundamental concepts of immunization have been around for over a thousand years, beginning with the practice of variolation: exposing healthy individuals to a small amount of pathogen in order to build immunity to a disease. The breakthrough discovery, however, came in 1796, when Edward Jenner used material from cowpox pustules to immunize humans against smallpox.
Modern vaccine development employs a variety of techniques to design and manufacture an effective product, relying on genomics, structural biology and a thorough understanding of the pathogen. Despite technological advancements, conventional vaccine development methods face significant challenges.
DNA or RNA?
Conventional vaccine development takes time. It can be as long as 10–15 years from initial research to market availability (1). Protein antigens or pathogens are manufactured in dedicated fermentation or cell culture setups, and this specialized equipment may require considerable capital investment that incurs significant financial risks for the manufacturer (2). Most notably, this process is unsuited to situations that demand a rapid response—such as a global pandemic.
A promising alternative to conventional vaccine development is the use of nucleic acid (DNA or RNA) in vaccines. The concept is not new. In 1990, a pioneering experiment showed that direct injection of DNA or RNA into mouse muscle tissue resulted in endogenous expression of the encoded gene (3). The manufacturing process for nucleic acids is comparatively less complex than that for proteins or pathogen cultures, and it can be rapidly scaled as needed. Since the same process can be used regardless of the encoded antigen, the same manufacturing facility can be used to produce multiple vaccines, cutting production time and costs (2).
Both DNA and RNA vaccines result in the production of the antigen within the body’s own cells, in much the same way that would occur during an actual viral infection. However, since the vaccine only encodes a viral protein (or a protein subunit) and not the full viral genome, there is no danger of an actual viral infection. In 1998, the first human trial of a DNA vaccine against human immunodeficiency virus type 1 (HIV-1) was reported (4). Since that time, DNA vaccines have been developed against a range of pathogens, including hepatitis B, influenza, Ebola and Zika viruses (reviewed in 5). However, there are currently no DNA vaccines licensed for human use.
Why mRNA?
DNA vaccines face two potential barriers: they must first cross the cell membrane and then enter the nucleus, where the DNA is transcribed into mRNA. Next, the mRNA is transported out of the nucleus into the cytoplasm, where the cellular translational machinery synthesizes the corresponding protein antigen. mRNA vaccines offer a shortcut to this process, because they are directly translated in the cytoplasm. (See How Does an mRNA Vaccine Work?) These factors also make the required dose much smaller for mRNA vaccines: up to 1,000 times lower than a comparable DNA vaccine (2).
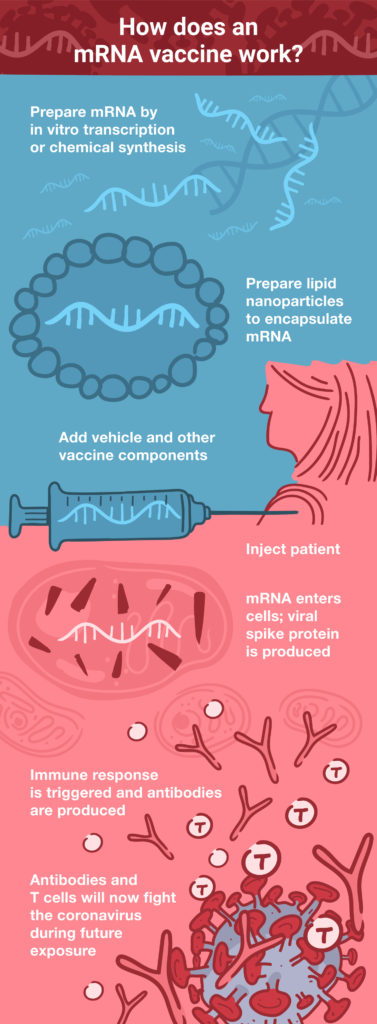
During the early development of DNA vaccines, one of the concerns was the possibility of the foreign DNA integrating into the host genome. Although this concern has largely been mitigated with advancements in the development of DNA viral vectors, it is completely absent with mRNA vaccines that never enter the nucleus and have a much shorter half-life within the cell, compared to DNA.
RNA vaccines do have their downside, however. To begin with, RNA is less stable than DNA. This is one reason why mRNA vaccine formulations require specialized, cold shipping and storage conditions: the Pfizer/BioNTech vaccine will be shipped on dry ice (–80°C), while the Moderna vaccine will be shipped at a more manageable –20°C using gel packs. Upon receipt, the vaccines can be stored in a conventional refrigerator for short periods of time.
Once the RNA is injected into the body, it faces another challenge. Our cells have evolved elaborate defense mechanisms intended to destroy foreign, unprotected or “naked” RNA. However, the susceptibility of mRNA to degradation can be reduced by modifying the RNA during synthesis. One modification is to add in nucleoside analogs that resemble the normal nucleosides found within RNA (A, U, C and G) but have minor structural changes that make the RNA more resistant to degradation by ribonucleases. Additional structural modifications and the inclusion of regulatory sequences can also improve the stability of mRNA (6).
Finally, the mRNA can be delivered in the form of a complex with lipid nanoparticles, which helps to stabilize the mRNA, makes it easier to enter the cell, and increases the amount of antigen produced per cell (7). Lipid nanoparticle formulations also elicit a stronger immune response compared to naked mRNA (8).
With DNA vaccines, many copies of mRNA can be produced from a single DNA template once it reaches the nucleus within the cell. This results in a higher yield of antigen per cell, compared to an mRNA vaccine. Recent methods to create self-amplifying mRNA vaccines have compensated for this shortcoming (reviewed in 9). It is not clear if either the Pfizer/BioNTech or Moderna vaccines use this strategy, since details about the mRNA structure have not been released.
The recent coronavirus vaccine announcements have fueled hope for a return to normalcy. Ultimately, however, effective vaccines are only one part of a comprehensive pandemic response strategy. There remains an urgent need to develop effective therapeutic agents to treat those already infected. Meanwhile, measures to reduce the risk of infection, such as masks and social distancing, remain the first line of defense.
Learn more about viral vaccine development with our SARS-CoV-2 Research, Vaccine and Therapeutic Development resource page.
References
- The complex journey of a vaccine. International Federation of Pharmaceutical Manufacturers & Associations (IFPMA). [https://www.ifpma.org/resource-centre/the-complex-journey-of-a-vaccine-final/; last accessed November 24, 2020]
- Rachlin, E. and Watson, M. (2017) White paper: mRNA vaccines: disruptive innovation in vaccination. Moderna Therapeutics. [www.modernatx.com; last accessed November 24, 2020]
- Wolff, J.A. et al. (1990) Direct gene transfer into mouse muscle in vivo. Science 247 (4949), 1465–1468.
- MacGregor, R.R. et al. (1998) First human trial of a DNA-based vaccine for treatment of human immunodeficiency virus type 1 infection: safety and host response. J. Infect. Dis. 178(1), 92–100.
- Rauch, S. et al. (2018) New vaccine technologies to combat outbreak situations. Front. Immunol. 9, 1963.
- Holtkamp, S. et al. (2006) Modification of antigen-encoding RNA increases stability, translational efficacy, and T‑cell stimulatory capacity of dendritic cells. Blood 108, 4009–4017.
- Geall, A.J. et al. (2012) Nonviral delivery of self-amplifying RNA vaccines. Proc. Natl Acad. Sci. USA 109, 14604–14609.
- Lutz J. et al. (2017) Unmodified mRNA in LNPs constitutes a competitive technology for prophylactic vaccines. NPJ Vaccines (2017) 2, 29.
- Fuller, D.H. and Berglund, P. (2020) Amplifying RNA vaccine development. N. Engl. J. Med. 382, 25.
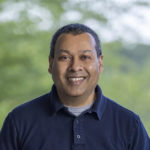
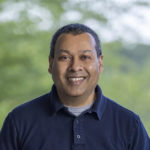
Latest posts by Ken Doyle (see all)
- Will Artificial Intelligence (AI) Transform the Future of Life Science Research? - February 1, 2024
- RAF Inhibitors: Quantifying Drug-Target Occupancy at Active RAS-RAF Complexes in Live Cells - September 5, 2023
- Synthetic Biology: Minimal Cell, Maximal Opportunity - July 25, 2023
Hello I am fascinated by the concept of mRNA vaccines. However I am not a scientist. Just a curious citizen interested in immunology. My question is, if people who are having severe reactions to COVID-19 (in laymen’s terms cytokine storm) which is really an OVER reaction of their immune system, then how does the vaccine prevent one with an autoimmune disorder from developing an even worse immune result? Wouldn’t the better option be to nix the vaccine and identify through DNA testing who is most likely to have an overreaction to the virus and then give them immune suppresant therapy? Can the current vaccines offered do both? Both currectly suppress the immune system to prevent cytokine storm and at the same time produce antigens to protect against the virus itself? Could people with overactive immune systems develope autoimmune disorders from the vaccines? Is this a risk? Thank you so much and I wish you all the best in your amazing work towards research of preventative measures during this pandemic.
Jennifer Rowse’s comment of 1/26/21 was very insightful. Her concluding concern regarding whether or not people with overactive immune systems could develop autoimmune disorder some time after receiving mRNA vaccine was in my opinion, a valud one. Can we look forward to getting a response to it? If so, approximately when and where should we keep a lookout for it?
@Jennifer Rowse: Those are great non-scientist questions. For Covid-19, I noticed that people who have cytokine storms cannot simply be described as having strong immune systems. Instead, they are not the yound people whose immune systems are most active. Instead, most of these people have some conditions where oxidative stress plays a role. Therefore, while cytokine storm may not help, vaccines could help, because the vaccined do not contain “live” viruses which can replicate and kill human cells. I know my answer is not ideal, but this is just to give you some information that I know. I have a biomedical background (toxicology), highly concerned and checked a lot of COVID-19, but I myself am not a viralogist or immunilogist.
We thank you for your comments and curiosity. In writing this article we enjoyed researching the (scientific) literature on this topic. While we will not comment on any specific therapies or treatment options, we do encourage your continued reading of credible scientific resources.
Informative article as well as intelligent comments. It is great to have a forum that facilitates open, honest discussion.
Whilst it may seem naive, I firmly believe that the best defense to diseases us a robust immune system in a healthy body. Disease cannot flourish if the terrain is healthy, ie inhispitable to pathogens.
I am not anti-vax in any way, but often wonder about how effective it really is.
Many people have not been unvaccinated since childhood, yet do not catch any of these ‘communicable’ diseases like ‘flu, measles, covid, etc. These are healthy people across all age groups, who have exemplary lifestyle habits.
Perhaps taxpayer funds would be better spent on helping people maintain a healthy lifestyle, rather than on managing chronic ‘dis-ease’ through the lifelong use of pharmaceuticals and the attendant adverse effects.
As for the current pandemic – a virus is so small that few, if any, masks could block it. I also doubt that lockdowns can stop a virus, which is aerosolized and does not need vehicles to travel. Unlike bacteria, a virus needs a host, eg a cell, in order to replicate.
Please share some articles on healthy lifestyles.
Sylvia, prevention is almost always more cost-efficient than treatment. Therefore, I agree with your overrall great ideas. For Covid-19, it actually fits into this big picture, since the mortality rate among the young and healthy is lower. However, for people who are at the same age, overall health status is just one factor in deciding the outcome, i.e., how serious the symptoms will be. Other factors, such as genetic profiles, play a big role, too. But at this time, there is no easy way to tell which patients are going to be fine or die based on genetics, even if the whole genome information is collected. Therefore, vaccine can help, not so much in stopping transmission, but in preventing the vast majority of hospitalization chance. Plus, though the virus is small, the droplets from Covid patients are large enough to be blocked by masks. This is also why all epidemiologists emcourage the use of masks. There is a study from Pittsburgh showing the effectiveness of the surgical mask in lowering flu transmission. Masks work two ways, not just protecting others, as the CDC had wrongly (and surprisingly) described in early 2020.