Imagine you’re taking a refreshing night swim in the warm blue waters of Vieques in Puerto Rico. You splash into the surf and head out to some of the deeper waters of the bay, when what to your wondering eyes should appear, but blue streaks of light in water that once was clear. Do you need to get your eyes checked? Are you hallucinating? No! You’ve just happened upon a cluster of dinoflagellates, harmless bioluminescent microorganisms called plankton, that emit their glow when disturbed by movement. These dinoflagellates are known to inhabit waters throughout the world but are generally not present in large enough numbers to be noticed. There are only five ecosystems in the world where these special bioluminescent bays can be seen, and three of them are in Puerto Rico.
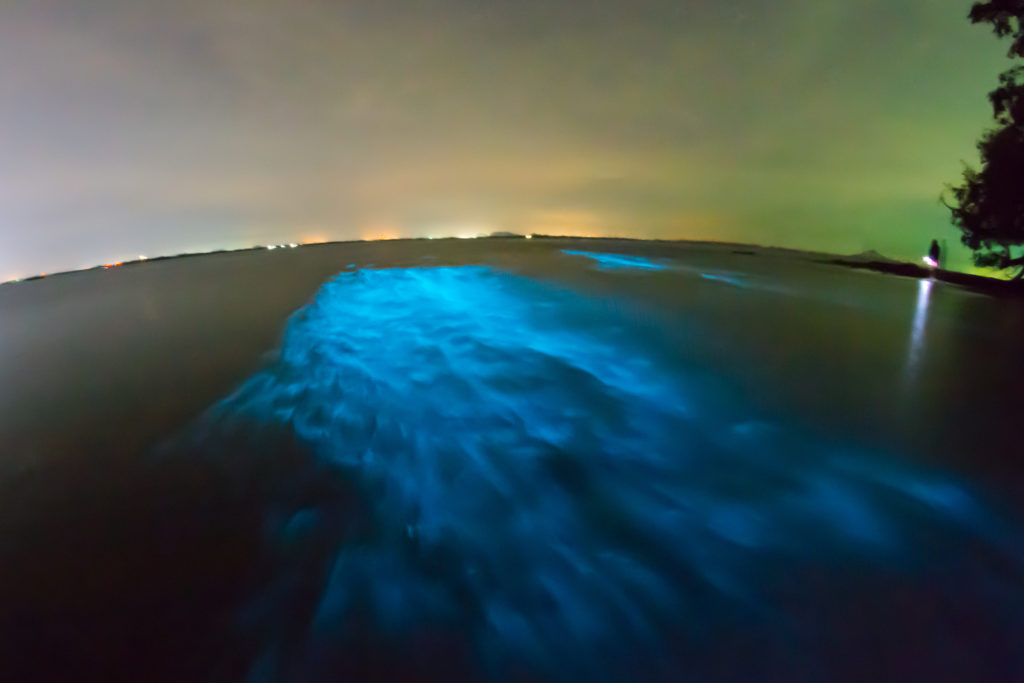
But you don’t have to travel to Puerto Rico or swim with plankton to see bioluminescence. There are bioluminescent organisms all over the world in many unexpected places. There are bioluminescent mushrooms, bioluminescent sea creatures—both large and small (squid, jellyfish, and shrimp, in addition to the dinoflagellates)—and bioluminescent insects, to name a few. Bioluminescence is simply the ability of living things to produce light.
Simply isn’t really the right word, however. The process that these organisms use to create light while not interfering with life itself is complex and fascinating. Take fireflies, for instance—they can make unique patterns of light by bringing air into their abdomens in the presence of a chemical called luciferin, which interacts with another molecule, an enzyme called luciferase that allows the firefly to create flashes of light. These are natural compounds that bioluminescent organisms produce within their own cells.
Why is it called luciferase? Typically, biological molecules that speed up naturally occurring chemical reactions are enzymes, and scientists use the suffix “-ase” as common nomenclature for these types of proteins. For this molecule in particular, the Latin root of the name “lucere” means “to shine”.
The luciferase reaction also involves other components, such as magnesium ions and ATP (the energy stores within cells), which are also present in the cellular environment. Cool, right? LITERALLY. Bioluminescent light is very energy efficient: very little of the energy produced in the light-producing reactions that create bioluminescence is lost as heat, which is important because otherwise it could burn the very cells that produce the compounds that make the light.
How does luciferase help produce bioluminescence?
Luciferase is an enzyme, a protein that acts as a chemical reaction catalyst, allowing luciferin, ATP, and other cellular ions to create light. The chemical reaction itself involves two steps – in the presence of luciferase, luciferin + ATP make luciferyl adenylate + ADP. The next step is that the luciferyl adenylate + oxygen is changed into oxyluciferin + AMP and of course, light.

This article from How Stuff Works, 2006 is another great resource for learning more about the luciferase reaction.
Why do organisms use bioluminescence?
The creation of light in living organisms is thought to benefit each species for different reasons. Current estimates are that about 1,500 different species can produce light using bioluminescence, and more are being discovered everyday. Here are some interesting ways in which some of these species use bioluminescence:
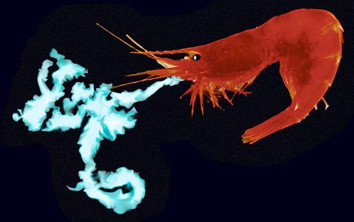
- Squid, which live deep in the ocean use bioluminescence to help them see. They have photophores along their body produce a deep blue light, which work in conjunction with the squid’s highly developed eyes and allow them to more easily find prey.
- In a sneakier approach to finding a meal, the anglerfish has a symbiotic relationship with bioluminescent bacteria. Anglerfish live deep in the ocean, impenetrable by light. As their name implies, this species ‘fishes’ for their meal by dangling a bulb above their sharp-toothed mouths. This bulb is filled with bioluminescent bacteria, and the light attracts other sea life, which the anglerfish is quick to snap up.
- Fireflies use bioluminescence to communicate. Different kinds of fireflies communicate using different flash patterns and some even coordinate their flashes to have greater success in mating. Research has shown that in some species, females prefer males that can flash brighter, longer signals. So, why haven’t males evolved to be super-flashers? Well, it turns out that some females mimic other species’ signals so that they can attract males and rather than mate with them, actually kill and eat them!
- How might a mushroom benefit from an unearthly glow? Already a bit weird-looking, a glow in the dark mushroom is a sight to behold. While many mushroom caps glow to attract animals, which then spread the fungi’s spores and allow more mushrooms to bloom, others have glowing mycelium – the intricate root system mushrooms use to feed, grow, and communicate. Scientists think that mushroom mycelium might glow for the opposite reason – to dissuade animals from disturbing them.
- Krill, described by National Geographic as “the fuel that runs our oceans” can produce a strong bluish light. These tiny shrimp-like creatures are the are the base of the food chain and are a main food source for whales, which can eat up to four tons of these tiny crustaceans a day! Krill, like dinoflagellates, only glow when disturbed. They live in gigantic schools. Scientists thus hypothesize that krill luminescence might serve as a sort of alarm when predators are near.
How can we use luciferase to advance science?
Biotechnology can be defined in one sense as using the wisdom of nature to improve the human condition. The ways in which bioluminescence is used in nature inspires our work as scientists. It’s extremely beneficial to be able to track various molecules within cells or systems that we are studying that would otherwise be invisible. When a molecule is used as a tracker, or reporter, within a living system we describe this molecule as a biomarker. Biomarkers don’t always have to be visible, but they do have to be detectable. Natural biomarkers are often hard to discover and characterize. Many years of research on these molecules are needed before they can be used for diagnostic purposes.
Biotechnology can be defined in one sense as using the wisdom of nature to improve the human condition.
But, as the Wild Kratts say, what if we could engineer the perfect biomarker? What if we could put the gene for luciferase into the system we are studying and be able to track our results using light?
As it turns out, we can – and we do! Scientific work has led to numerous applications for bioluminescence as a biomarker. We can study what genes are expressed and when – for example to determine which genes are associated with disease, or differences between different types of cells (cardiac vs. skin, kidney vs. neuron, stem cell vs. brain cell), whether cells are living or dying, and many other biological processes, all by tracking light!
Using luciferase to study when and where genes are expressed
In the case of gene expression, researchers can study how much of a particular protein of interest is made by engineering the gene associated with this protein to express luciferase (or another reporter marker) as well. Then, luciferase can be detected, and the amount of light output is a proxy for the protein of interest. A common way to do this would be to insert the reporter gene downstream of a promoter within an expression vector, perform genetic transformation to create millions of copies of this engineered construct, and then transfect the engineered DNA into cultured cells which would then allow these cells to produce luciferase along with the protein of interest.
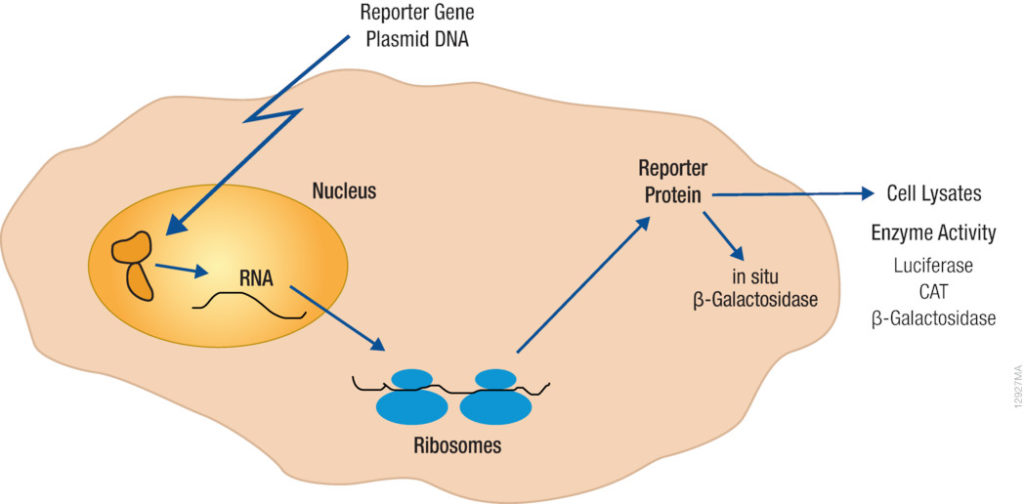
This type of work is central basic molecular biology research. In addition to giving us a sense of how much protein is made, luciferase can also show us where it is made, giving us visual indicators of where proteins of interest are localized within the cell. Here is a two-minute video that illustrates this concept:
Using bioluminescence to investigate cell health and monitor the impact of therapeutic compounds
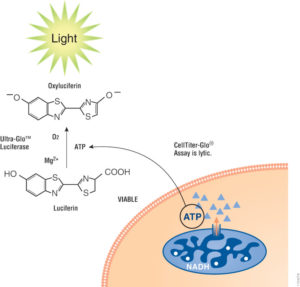
We can also use luciferase to investigate cell health and monitor the impact of drug treatments. For example, chemotherapy is intended to kill cells – particularly the rapidly dividing cells associated with cancer. We can seed cancerous cells in small culture wells or test wells, and treat them with chemotherapeutic candidate drugs. As a control, the same cells are grown under similar conditions, but left untreated. To determine if the treatment drug is effective, researchers need to test to see whether cells are dying as would be expected or thriving, which would mean the drug treatment is not working. Since counting all the cells present in each test well would not be possible, researchers can instead rely on the amount of light given off by the cells within the well as an indication of how many cells are producing ATP, a hallmark of living cells. If we compare the light given off by cells in treated versus untreated wells, where light is a proxy for the number of live cells, the amount of light given off by the untreated samples should be higher than the treated wells provided the chemotherapeutic is effective.
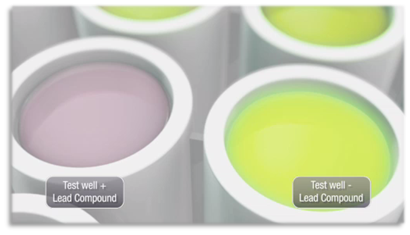
Summary
There are many other ways in which the phenomenon of bioluminescence can be used within biological systems to give us information about what is happening within these systems, including but not limited to monitoring water systems to ensure high quality drinking water, in-vivo imaging monitor things like circadian rhythms, as well as for detecting proteins, if a blood sample contains antibodies to COVID-19 or even vaccine research and development.
The video below gives a more detailed explanation of some of the ways luciferase assays can answer biological questions
Scientists continue to be inspired by nature and the amazing phenomena present in the world around us, and we hope that you are, too!
Related Posts
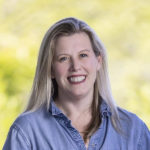
Amy Prevost
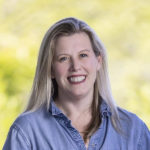
Nice article Amy! Just a quick question: where did you read, that there are only five bioluminescent ecosystems in the world or how do you define that? By a quick google search a found many more than five places with that phenomenon. Looking forward to your reply. Thank you!
https://triponzy.com/blog/bioluminescent-beaches-in-the-world/
https://www.oyster.com/articles/7-bioluminescent-beaches-and-bays-that-glow-at-night/
Hi Clara, The source that is linked from the post actually specifies five places with a high enough concentration to be considered “bioluminescent bays”, that is probably some kind of special designation. I have updated the post to reflect this. Thanks for asking. Michele