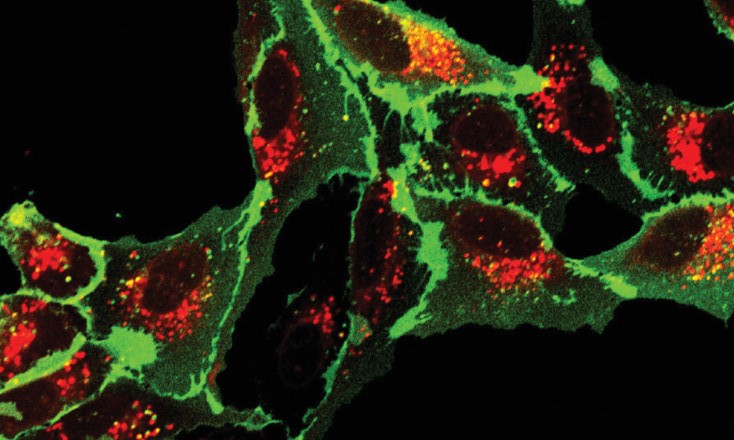
Fluorescent tags (fluorophores), have become excellent tools for labeling cells and cellular components. They can be used for imaging large molecules like proteins, on down to cellular components and enzymes such as transcription factors. Once labeled, these molecules can be tracked in tissue or inside a cell, when the right tag is used.
What is the ‘right’ tag? It’s a tag with bright signal, with low background and good photostability. For small cell components like organelles, the tag must be cell-permeable and small enough to not interfere with normal cellular processes such as transcription and metabolism.
Significant advances have been made in fluorescent tags in the past two decades. Here we look at several papers noting these advances.
Wysocki and Lavis (2011). Advances in the chemistry of small molecule fluorescent probes. Current Opinion in Chemical Biology, 15, 752–9.
This review looks at the state of small molecule fluorophores in 2011, noting the importance of these tools in advancing the study of cellular events. The authors used chemical modifications to activate the fluorophore, creating brighter synthetic dyes, which aided in regulating placement and timing of fluorescence.
For example, palladium-catalyzed reactions, widely used at this time in medicinal chemistry, were starting to be applied to dye synthesis, to create libraries of rhodol compounds. In another example, palladium was used to change a hydroxycoumarin compound to the isologous aminocoumarin, resulting in a dye with good fluorescence over a wide range of pH values, ideal for use in the cellular microenvironment.
Core structural elements of fluorescent dyes have been chemically manipulated and used to study structure-activity relationships. Screening these dyes in live cells helped to identify their cellular specificity. And combinatorial chemistry techniques resulted in dyes with tunable emission wavelengths.
The authors discussed fluorescent substrates that can be masked with enzyme labile groups, allowing the fluorescent probe to be localized and fluorescence to be regulated with endogenous or exogenous proteins, creating an off/on switch. Improving stability and local distribution of the released fluorophore limits background fluorescence and improves the ability to identify enzymatic activity spatially.
Chemical stability of fluorogenic enzyme substrates is important for biological imaging. For example, Wysocki et al. substituted acetate esters on fluorescein with acetoxymethyl ester, replacing an unstable phenolic ester with a stable alphatic ester, using a spacer that decomposes upon ester cleavage.
Use of these tools to successfully image targeted proteins in live cells is the ultimate goal. The early 2000s saw development of self-labeling protein tags, such as SNAPTag and HaloTag. Chemical modification of these protein tags can improve their use in labeling by adding the ability to fluorescently activate them and improve their cell membrane permeability.
In summary, Wysocki and Lavis predicted further chemical approaches to control positioning and activation of fluorophores, improving their use in biological research.
Presman, D.M. et al. (2017) Quantifying Transcription Factor Binding Dynamics at the Single-molecule Level in Live Cells. Methods 123, 76–88.
In this paper, the glucocorticoid receptor was used to study transcription factors by single molecule tracking (SMT).
When discussing the choice of a fluorophore, the authors note that while live-cell imaging was revolutionized by discovery of genetically-encoded fluorophores like GFP, these proteins have poor brightness and photostability and are thus not ideal for potentially long chromatin binding events. Furthermore, Alexa Fluor, Cy and ATTO conjugated to proteins would be difficult to introduce to cells, requiring microinjection of individual proteins.
The authors instead choose to work with chemical fluorophores of the self-labeling tag type, such as HaloTag.
HaloTag is a bacterial dehalogenase enzyme variant that reacts rapidly and specifically with chloroalkane ligands. HaloTag is engineered so that the catalytic process stops at an ester intermediate, allowing HaloTag to be used to covalently label the protein being studied. SNAP-tag and CLIP-tag are based on the same principle and are complementary to HaloTag, allowing multicolor imaging.
The authors considered whether the fluorophore label and tagging system affects resulting data and introduces bias. To answer this question they compared labeling with Janelia Fluor (JF549) ligand to HaloTag, SNAP-tag and Click-tag systems using the glucocorticoid receptor as a model transcription factor.
Regarding photostability of JF549 with the various systems, they found the HaloTag-JF549 conjugate to have better photostability than the JF549-SNAP-tag or JF549-Click-tag systems.
They demonstrated using single-cell studies, that transcription factors interact with chromatin surprisingly dynamically.
Meissner, G.W. et al. (2018) Optimization of fluorophores for chemical tagging and immunohistochemistry of Drosophila neurons. PLOS ONE 13, e0200759.
Meissner et al. explored the optimization of fluorophores for chemical tagging and immunohistochemistry of neurons in Drosophila melanogaster. The study builds on the existing self-labeling tag techniques that use small molecule ligands to bind covalently to genetically encoded enzyme-based tags. This method, known for quicker processing and reduced background staining compared to traditional immunohistochemistry (IHC), was employed to overcome common issues like poor tissue penetrance and high background staining associated with conventional antibody-based labeling.
The study introduced four new fluorophore ligands based on Cy, Janelia Fluor, Alexa Fluor and ATTO dyes. These ligands were designed to be compatible with recently improved Drosophila transgenes and showed improved labeling intensity, demonstrating a significant advancement over first-generation chemical tag reporters.
The authors showed that using DPX (a xylene-based mounting medium) for tissue clearing significantly enhanced the signal quality when non-cyanine fluorophores are used, facilitating clearer, more distinct neuronal structure imaging.
Meissner et al. successfully combined the chemical tagging with standard immunohistochemistry techniques. This approach leverages the specificity and rapid labeling of chemical tags with the robustness of antibody-based detection, offering a comprehensive toolkit for detailed neuronal studies.
Additionally, they made a series of methodological enhancements and comparisons:
- The new fluorophores were synthesized and evaluated for their effectiveness in labeling fixed tissue, specifically focusing on their brightness and stability.
- The newly developed tags were directly compared to traditional IHC methods, noting improvements in speed and signal-to-noise ratio.
- The utility of the new methods was validated in practical experimental setups, including detailed neuronal mapping in Drosophila brains, highlighting the practical benefits and potential for broad application in neuroscience research.
In conclusion, these studies marked a significant step forward in the field of neuronal imaging and labeling. By optimizing fluorophores for chemical tagging and integrating these with traditional IHC methods, they developed a more efficient and robust approach for studying neuronal architecture. This work not only enhanced the ability to visualize neuronal circuits in model organisms like Drosophila but also set the stage for additional innovations in fluorescence-based imaging technologies.
Hensel et al. (2024) Rapid in-EPON CLEM: Combining fast and efficient labeling of self-labeling enzyme tags with EM-resistant Janelia Fluor dyes and StayGold. Heliyon 10, e28055.
In March of 2024, Hensel et al. published a study introducing a streamlined and efficient approach for in-resin correlative light and electron microscopy (CLEM) by integrating self-labeling enzyme tags with EM-resistant Janelia Fluor® dyes and the StayGold fluorescent protein. This combination aims to address the challenges of preserving fluorescence during the harsh conditions of electron microscopy (EM) sample preparation.
Janelia Fluor® dyes are specifically engineered for increased brightness and photostability, making them suitable candidates for CLEM workflows. The study evaluated various Janelia Fluor® dyes, including JF479, JF525, JF549, JFX549, JFX554, JF585, JF646, JFX646 and JFX650, for their ability to retain fluorescence after conventional embedding (CE) processes involving osmium tetroxide fixation, dehydration and EPON embedding.
They found that:
- JFX549 and JFX554 dyes had the best fluorescence retention, retaining nearly 20% more fluorescence in EPON sections compared to the traditional TMR (tetramethylrhodamine) dye. These dyes provided consistent and reproducible fluorescence signals, facilitating precise correlation between light microscopy (LM) and electron microscopy (EM) images.
- JF549 also retained fluorescence but was more susceptible to minor variations in EM sample preparation.
- JF479, JF585, and the far-red dyes (JF646, JFX646, JFX650) failed to retain fluorescence effectively.
In conclusion:
- The study successfully demonstrated dual-fluorescence CLEM using combinations of HaloTag and SNAP-tag, or HaloTag and StayGold, which allowed simultaneous visualization of different cellular structures.
- The green fluorescent protein StayGold, known for its photostability, retained fluorescence in resin, enabling dual-fluorescence CLEM without the need for special adaptations to the workflow.
- The research highlights the potential for rapid, efficient labeling and imaging in CLEM workflows, making it accessible for routine use in various laboratories.
- The approach can be particularly beneficial for studying the ultrastructural context of labeled proteins within cells, facilitating advancements in cellular and molecular biology research.
This study identifies JFX549 and JFX554 as the most promising Janelia Fluor dyes for in-resin CLEM due to their superior fluorescence retention and stability. The combination of these dyes with self-labeling enzyme tags and the StayGold protein offers a robust and versatile method for high-precision correlative microscopy, enhancing the capability to visualize cellular structures in detail.
Summary
These papers, spanning 2011–2024, provide a look at developments in and use of HaloTag® and Janelia Fluor® ligands. The papers cover the period when chemical modifications of fluorescent dyes were proposed, through a period of dye improvements via chemical modification, allowing their use in deep cellular exploration, to expansion into new research areas due to dye improvements.
What do you think should come next?
The HaloTag® Janelia Fluor® Dyes are our brightest, most capable fluorescent dyes. Providing enhanced brightness in confocal imaging, these dyes were designed for super resolution techniques. We offer HaloTag Janelia Fluor ligands that cover the full spectrum of visible light.
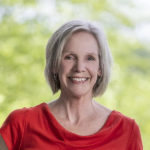
Kari Kenefick
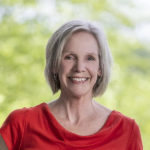
Latest posts by Kari Kenefick (see all)
- Base Editing Brilliance: David Liu’s Breakthrough Prize and Its Impact - May 28, 2025
- Neurons’ Role in FBP2 Regulation - October 24, 2024
- Fluorescent Ligands in Biological Research: Where We’ve Been, Where We’re Headed - June 27, 2024