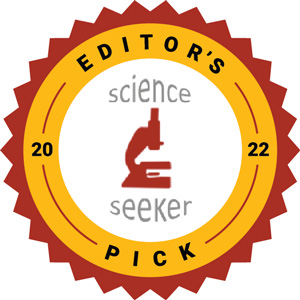
We’re entering the third year of the global COVID-19 pandemic, and it’s far from over. There has been considerable progress with SARS-CoV-2 vaccine development, with most of the focus on mRNA vaccines and adenoviral vector vaccines. Meanwhile, novel vaccine delivery systems are being tested among efforts to develop a “pan-coronavirus” vaccine that is effective against multiple variants. One such example is ferritin nanoparticle technology developed by researchers at the Walter Reed Army Institute of Research and their collaborators. Encouraging results from nonhuman primate studies, using several SARS-CoV-2 antigens, were published in 2021 (1–3).
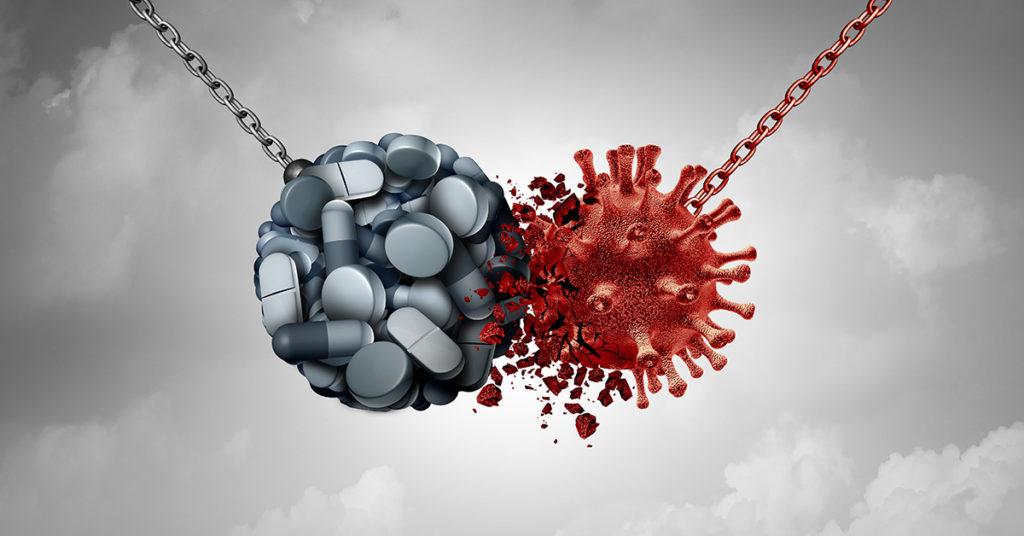
The current surge in COVID-19 cases that began last month is largely due to the Omicron variant in the US, according to data from the US Centers for Disease Control and Prevention (CDC). At present, we still don’t know enough about this variant, but it’s clear that its rapid spread is forcing us to re-examine what we know about SARS-CoV-2 (4). As the virus continues to mutate, new variants will continue to emerge and spread. Although current vaccines can provide protection against multiple variants, breakthrough infections are a concern. Vaccination is still the best option to reduce the risk of infection, hospitalization, and death compared to unvaccinated people.
It’s clear that vaccines are only part of an effective response to fighting the pandemic. Along with continued vaccine development efforts, attention must also be given to antiviral drug development for people already infected with COVID-19. Due to the lengthy process for new drug development, early efforts focused on repurposing existing drugs.
Remdesivir Revisited
Remdesivir was the first repurposed drug granted emergency use authorization (EUA) by the US Food and Drug Administration (FDA) for use in patients hospitalized with COVID-19. It is a nucleoside analog prodrug: when converted to its active form within infected cells, remdesivir competes with one of normal building blocks of viral RNA—adenosine triphosphate (ATP). The binding of active remdesivir to the viral RNA-dependent RNA polymerase (RdRP) inhibits RNA replication, thereby reducing the amount of virus particles in the cell. RdRp is a vital enzyme for viral replication and its structure is highly conserved, making it an attractive target for antiviral therapy that could target a range of viral variants.
Currently, there are over 100 clinical trials of remdesivir for COVID-19 listed in the clinicaltrials.gov database. The effectiveness of remdesivir appears to vary. A meta-analysis of randomized clinical trials published in 2021 showed little or no effect on all-cause mortality and uncertain effects on clinical improvement or worsening of COVID-19 symptoms (5). A more recent meta-analysis of hospitalized COVID-19 patients in India concluded that remdesivir did improve clinical outcomes, especially in patients <60 years old (6). As more results emerge from ongoing clinical trials, we should obtain more clarity about the efficacy and safety of remdesivir in treating patients hospitalized with COVID-19.
Molnupiravir (Merck)
In December 2021, the FDA announced an EUA for molnupiravir, an oral antiviral agent from Merck. The EUA notice indicated the drug’s use for the treatment of mild-to-moderate COVID-19 in at-risk adults for whom alternative COVID-19 treatment options are not accessible or clinically appropriate. Treatment should begin within 5 days of symptom onset. One advantage that molnupiravir has over remdesivir is its route of administration: it’s given orally, as opposed to intravenously.
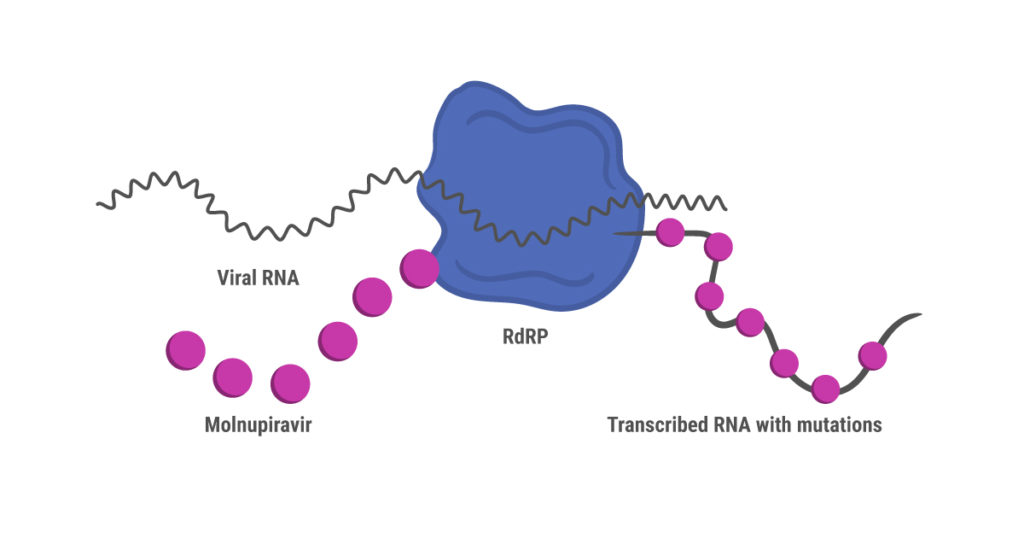
Like remdesivir, molnupiravir is a nucleoside analog prodrug that was originally developed to treat influenza (7). Unlike remdesivir, however, molnupiravir doesn’t shut down synthesis of the new viral RNA strand during replication. Instead, RdRP continues to synthesize RNA while introducing mutations due to misincorporation of nucleosides. These mutations accumulate over several cycles of replication, ultimately leading to death of the virus (8).
This mechanism of action has prompted concerns that molnupiravir might create a more virulent SARS-CoV-2 strain through random mutation, or even cause mutations in human RNA. These risks were assessed as being low before the drug received regulatory approval in the UK (7). Interim results from an international phase 3 clinical trial (MOVe-OUT) published in December 2021 showed that early treatment with molnupiravir reduced the risk of hospitalization or death through day 29, compared to the control group (9). Adverse events affected similar percentages of patients in the molnupiravir and control groups. However, further results were disappointing, and researchers have expressed concerns that that molnupiravir is not as effective as thought initially. A different, smaller trial reported phase 2 interim results showing that molnupiravir reduced nasopharyngeal SARS-CoV-2 viral loads (preprint, 10).
Paxlovid (Pfizer)
Prior to the molnupiravir EUA, in December 2021 the FDA issued an EUA for Paxlovid, another oral antiviral agent from Pfizer. The drug was recommended for treating COVID-19 in patients >12 years old at high risk for progression to severe symptoms, including hospitalization or death.
Paxlovid is a combination therapy that includes two drugs: PF-07321332 (nirmatrelvir) and ritonavir. Their mechanism of action is different from remdesivir and molnupiravir. When SARS-CoV-2 RNA is released into an infected cell, it uses the cellular translational machinery to make the proteins required for viral function and assembling new viruses. The new proteins are synthesized in a long, continuous “polyprotein”, after which two viral proteases cleave it into individual, smaller proteins.
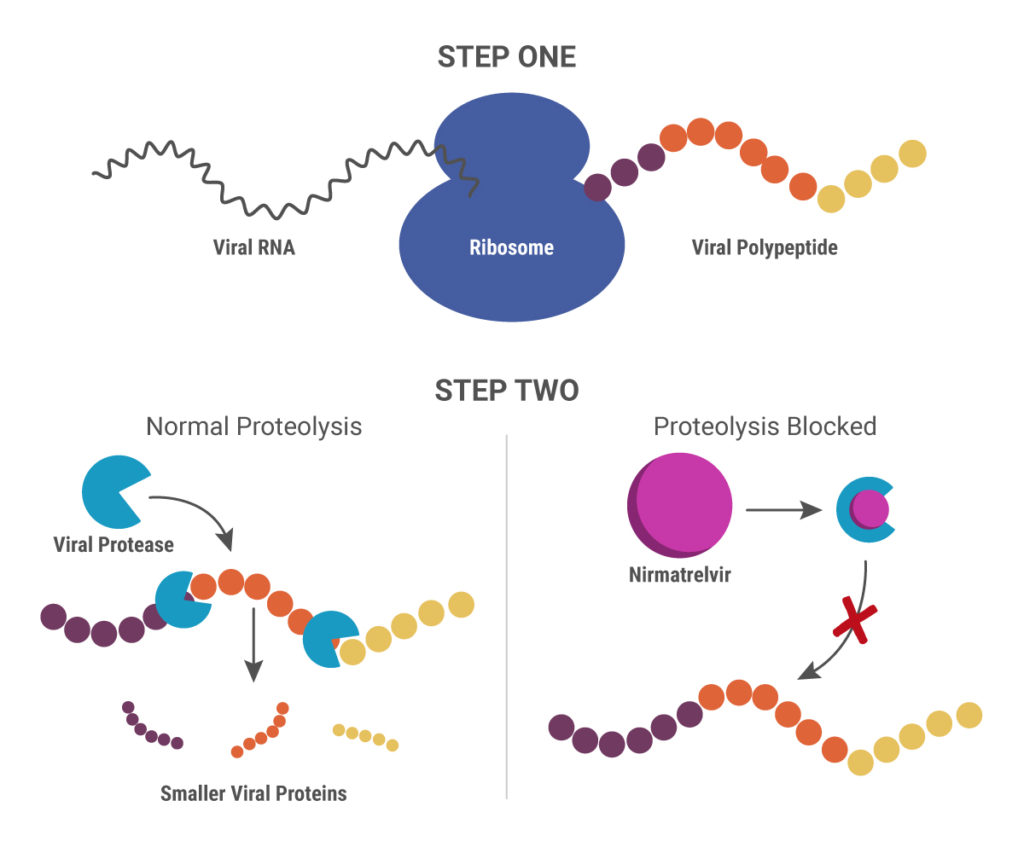
Nirmatrelvir blocks the action of one of these viral proteases, the 3-chymotrypsin-like protease (3CLpro), also known as the main protease (Mpro). Without the ability to produce functional proteins, the virus is unable to replicate. Ritonavir is another protease inhibitor originally developed to treat HIV-1 infections. In this scenario, it inhibits the cytochrome p450 enzyme CYP3A4 that breaks down nirmatrelvir, thereby boosting the action of nirmatrelvir (11).
Nirmatrelvir was designed specifically to target SARS-CoV-2 3CLpro, and it showed good pan-coronavirus antiviral activity during in vitro analysis, as well as in a mouse model system (12). Initial results from the phase 2/3 EPIC-HR clinical trial demonstrated that patients who received treatment within 3 days of showing COVID-19 symptoms had an 89% lower risk of hospital admission or death from any cause, compared to the placebo group (Pfizer press release). Final data from high-risk patients confirmed these results and were announced in another press release.
What’s Next?
As with any early-stage therapy, safety and efficacy data for both molnupiravir and Paxlovid will need careful review before the drugs are available for widespread use. Having an oral medication available to treat COVID-19, along with increased adoption of vaccines as a preventive measure, will be essential to stopping the spread of SARS-CoV-2.
Learn more about tools for COVID-19 therapeutic research with our SARS-CoV-2 Drug Discovery and Vaccine Development web page.
References
- Joyce, M.G. et al. (2021) SARS-CoV-2 ferritin nanoparticle vaccines elicit broad SARS coronavirus immunogenicity. Cell Rep. 37, 110143.
- Joyce, M.G. et al. (2021) A SARS-CoV-2 ferritin nanoparticle vaccine elicits protective immune responses in nonhuman primates. Sci. Transl. Med. DOI: 10.1126/scitranslmed.abi5735
- King, H.A.D. et al. (2021) Efficacy and breadth of adjuvanted SARS-CoV-2 receptor-binding domain nanoparticle vaccine in macaques. Proc. Natl. Acad. Sci. USA 118(38), e2106433118.
- Vogel, G. and Kupferschmidt, K. (2021) Early lab studies shed light on Omicron’s behavior. Science 374(6575), 1543.
- Ansems, K. et al. (2021) Remdesivir for the treatment of COVID-19 (Review). Cochrane Database Syst. Rev. 8, CD014962.
- Gupte, V. et al. (2022) Safety and clinical outcomes of remdesivir in hospitalised COVID‑19 patients: a retrospective analysis of active surveillance database. BMC Infect. Dis. 22, 1.
- Whitley, R. (2021) Molnupiravir—a step toward orally bioavailable therapies for Covid-19. N. Engl. J. Med. DOI: 10.1056/NEJMe2117814
- Malone, B. and Campbell, E.A. (2021) Molnupiravir: coding for catastrophe. Nat. Struct. Mol. Biol. 28, 706–711.
- Bernal, A.J. et al. (2021) Molnupiravir for oral treatment of Covid-19 in nonhospitalized patients. N. Engl. J. Med. DOI: 10.1056/NEJMoa2116044
- Fischer, W. et al. (2021) Molnupiravir, an oral antiviral treatment for COVID-19 (Preprint). medRxiv: DOI: 10.1101/2021.06.17.21258639
- Zeldin, R.K. and Petruschke, R.A. (2004) Pharmacological and therapeutic properties of ritonavir-boosted protease inhibitor therapy in HIV-infected patients. J. Antimicrob. Ther. 53, 4–9.
- Owen, D.R. et al. (2021) An oral SARS-CoV-2 Mpro inhibitor clinical candidate for the treatment of COVID-19. Science 374, 1586–1593.
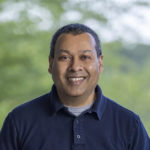
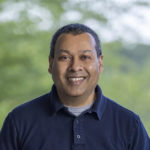
Latest posts by Ken Doyle (see all)
- Will Artificial Intelligence (AI) Transform the Future of Life Science Research? - February 1, 2024
- RAF Inhibitors: Quantifying Drug-Target Occupancy at Active RAS-RAF Complexes in Live Cells - September 5, 2023
- Synthetic Biology: Minimal Cell, Maximal Opportunity - July 25, 2023