This post is written by guest blogger, Amy Landreman, PhD, Sr. Product Manager at Promega Corporation.
Oxygen is necessary for animal life. It’s essential for cellular respiration and the production of energy (ATP) we require to survive. Given the need for oxygen, it isn’t surprising that our bodies have evolved ways to sense and adapt to decreased oxygen conditions (hypoxia). We can increase the production of new blood vessels by producing vascular endothelial growth factor (VEGF) or increase red blood cell (RBC) production by increasing the levels of eythropoietin (EPO), the hormone that plays a key role in the production of RBCs. But how does our body sense low oxygen, increase EPO levels, and kick our RBC production into gear? Nobel laureate Gregg L. Semenza has been honored for his contributions to our understanding of this process, and his research demonstrates the value of reporter genes and bioluminescence for studying gene regulation.
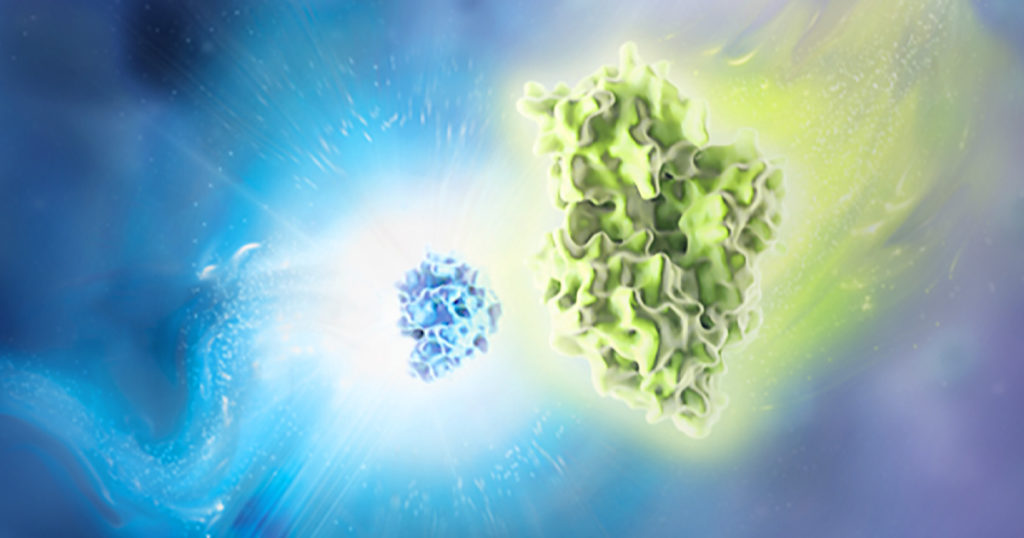