It is not difficult to appreciate why a keen sense of smell is important to well-being and to general living. While it signals the presence of delicious (or stale) food before we can even see or taste it, it has obvious great survival value to be able to alert living beings of danger such as certain poisons, leaking gas or fire. Humans are known to identify about 10,000 different types of odors. Of course dogs have vastly improved and keener sense of smell than human beings.
When odorant molecules (molecules that we can smell) reach the nostril, they dissolve in the mucus and bind to olfactory receptors present on the cilia of each sensory neuron. This binding activates a G-protein coupled cascade involving adenylyl cyclase. This causes the release of cyclic AMP and opening of cAMP-dependent sodium channels. Influx of sodium causes the membrane to depolarize and activate an action potential for propagation of the signal to the brain where it is analyzed and decoded(1). This seems pretty straightforward until one realizes the sheer magnitude of smells we are able to identify using this mechanism.
Genetically, mammals express 1,000 genes, mostly similar but varying slightly from each other in cells known as olfactory receptor cells, which occupy a small area in the upper part of the nasal epithelium. (Linda Buck and Richard Axel were awarded the Nobel Prize for discovery of olfactory receptor genes in 1991).
Researchers show that every single olfactory receptor cells expressed only one of the odorant receptor genes and each receptor can detect a limited number of odorant substances. Our olfactory receptor cells are therefore highly specialized for a few odors. As we know, most odors are very complex and contain many molecules that are capable of triggering responses. Each cell responds to more than one related odorant substances albeit with different intensities. So, we can now imagine a complex permutation and combinatorial code that forms a “pattern” that is registered in the brain. This explains how we are able to identify approximately 10,000 different odors.
Interestingly animals also “scout” the environment for olfactory cues by sniffing (similar to scanning the scene for visual cues). Animals can dynamically modify their odorant sampling by changing sniffing frequency and patterns (by modifying the air flow at which the odor stream is driven through the nasal passage) depending on whether the odorant molecule is present in high or low concentrations in the environment. Another astonishing fact is that the olfactory epithelium in vertebrate species has chromatographic properties. Therefore, molecules with different sorptive properties in the mucosa have different retention times and therefore trigger proportionately different magnitude neural responses. Consequently, mouse olfactory receptors are classified into two major groups- olfactory receptors that preferentially bind High-Sorptive (HS) or Low-Sorptive (LS) odorants. Further, the receptors that bind the LS molecules preferentially are present in areas that have low air flow or late in the air pathway.
Recent study by Roja-Libano and Kay(2) tested what is called the zonation hypothesis in waking animals, which proposes that animals manipulate the air flow through the nasal passage through sniffing patterns to direct the odorant flow to the zone in the olfactory epithelium where it would be recognized in the most efficient manner. They performed a very elegant series of experiments where they trained two groups of rats to detect HS and LS odorants in binary mixtures respectively. The behavioral paradigm was correlated with local field potential in the olfactory bulb via implanted electrodes. They also recorded the discrimination performance (ability to select the correct odorant in the binary mixture) based on the number of inhalations during odor sampling. Based on this study, they concluded that detection performance depended on target odorant sorptive property. Also, the LS group of rats showed longer sniffing sessions as if to sample the air for a longer time in order to identify the low target. As they learned to identify the target during the learning sessions, the sniffing bout duration decreased indicating that once they know how to adjust the airflow rate for the particular odorant, they do not have the necessity of a longer sniffing bout.
So, the next time your nose tells you there is a coffee shop nearby, think about how the physical properties of the odorant molecule in terms of its sorptive property, modulation of air-flow to adjust sniffing strategies work together to direct the molecule to its “zone” for effective binding to olfactory receptors and generation of a combinatorial code for coffee to enable subsequent identification of the coffee smell by the brain.
References
- Nakamura T. (2000) Cellular and molecular constituents of olfactory sensation in vertebrates. Comp Biochem Physiol A Mol Integr Physiol. 126, 17–32.
- Rojas-Líbano D, Kay LM. (2012) Interplay between Sniffing and Odorant Sorptive Properties in the Rat. J Neurosci. 2012 32, 15577–89.
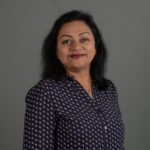
Anupama Gopalakrishnan
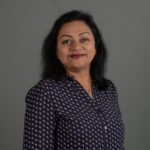
Latest posts by Anupama Gopalakrishnan (see all)
- Mitochondrial DNA Typing in Forensics - February 25, 2015
- PowerQuant System: Tool for informed casework sample processing decisions - July 21, 2014
- Biology of Overeating and the Weight-Gain Cycle - April 28, 2014
The 10,000 odors number comes from a popular article in Scientific American and can be traced back to some Japanese conference. There is no scientific evidence to back it up. It’s a myth.